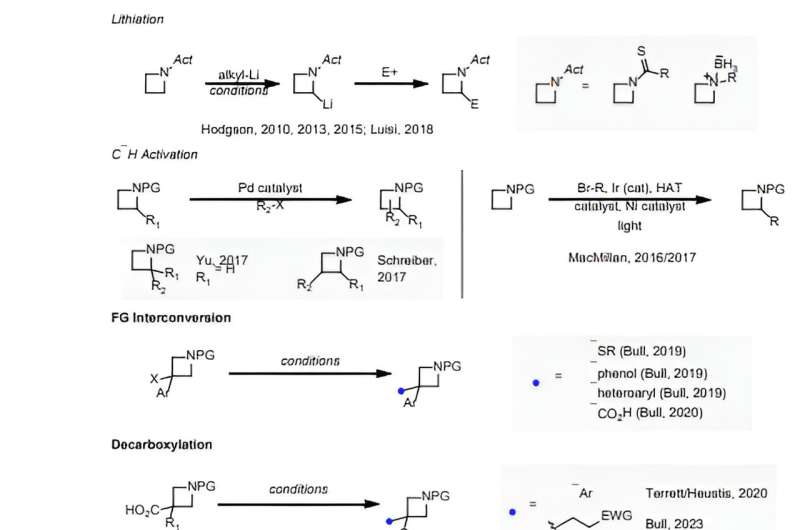
Researchers from MIT and the University of Michigan have discovered a new way to drive chemical reactions that could generate a wide variety of compounds with desirable pharmaceutical properties.
These compounds, known as azetidines, are characterized by four-membered rings that include nitrogen. Azetidines have traditionally been much more difficult to synthesize than five-membered nitrogen-containing rings, which are found in many FDA-approved drugs.
The reaction that the researchers used to create azetidines is driven by a photocatalyst that excites the molecules from their ground energy state. Using computational models that they developed, the researchers were able to predict compounds that can react with each other to form azetidines using this kind of catalysis.
“Going forward, rather than using a trial-and-error process, people can prescreen compounds and know beforehand which substrates will work and which ones won’t,” says Heather Kulik, an associate professor of chemistry and chemical engineering at MIT.
Kulik and Corinna Schindler, a professor of chemistry at the University of Michigan, are the senior authors of the study, which appears today in Science. Emily Wearing, recently a graduate student at the University of Michigan, is the lead author of the paper. Other authors include University of Michigan postdoc Yu-Cheng Yeh, MIT graduate student Gianmarco Terrones, University of Michigan graduate student Seren Parikh, and MIT postdoc Ilia Kevlishvili.
Light-driven synthesis
Many naturally occurring molecules, including vitamins, nucleic acids, enzymes and hormones, contain five-membered nitrogen-containing rings, also known as nitrogen heterocycles. These rings are also found in more than half of all FDA-approved small-molecule drugs, including many antibiotics and cancer drugs.
Four-membered nitrogen heterocycles, which are rarely found in nature, also hold potential as drug compounds. However, only a handful of existing drugs, including penicillin, contain four-membered heterocycles, in part because these four-membered rings are much more difficult to synthesize than five-membered heterocycles.
In recent years, Schindler’s lab has been working on synthesizing azetidines using light to drive a reaction that combines two precursors, an alkene and an oxime. These reactions require a photocatalyst, which absorbs light and passes the energy to the reactants, making it possible for them to react with each other.
“The catalyst can transfer that energy to another molecule, which moves the molecules into excited states and makes them more reactive. This is a tool that people are starting to use to make it possible to make certain reactions occur that wouldn’t normally occur,” Kulik says.
Schindler’s lab found that while this reaction sometimes worked well, other times it did not, depending on which reactants were used. They enlisted Kulik, an expert in developing computational approaches to modeling chemical reactions, to help them figure out how to predict when these reactions will occur.
The two labs hypothesized that whether a particular alkene and oxime will react together in a photocatalyzed reaction depends on a property known as the frontier orbital energy match. Electrons that surround the nucleus of an atom exist in orbitals, and quantum mechanics can be used to predict the shape and energies of these orbitals. For chemical reactions, the most important electrons are those in the outermost, highest energy (“frontier”) orbitals, which are available to react with other molecules.
Kulik and her students used density functional theory, which uses the Schrödinger equation to predict where electrons could be and how much energy they have, to calculate the orbital energy of these outermost electrons.
These energy levels are also affected by other groups of atoms attached to the molecule, which can change the properties of the electrons in the outermost orbitals.
Once those energy levels are calculated, the researchers can identify reactants that have similar energy levels when the photocatalyst boosts them into an excited state. When the excited states of an alkene and an oxime are closely matched, less energy is required to boost the reaction to its transition state—the point at which the reaction has enough energy to go forward to form products.
Accurate predictions
After calculating the frontier orbital energies for 16 different alkenes and nine oximes, the researchers used their computational model to predict whether 18 different alkene-oxime pairs would react together to form an azetidine. With the calculations in hand, these predictions can be made in a matter of seconds.
The researchers also modeled a factor that influences the overall yield of the reaction: a measure of how available the carbon atoms in the oxime are to participate in chemical reactions.
The model’s predictions suggested that some of these 18 reactions won’t occur or won’t give a high enough yield. However, the study also showed that a significant number of reactions are correctly predicted to work.
“Based on our model, there’s a much wider range of substrates for this azetidine synthesis than people thought before. People didn’t really think that all of this was accessible,” Kulik says.
Of the 27 combinations that they studied computationally, the researchers tested 18 reactions experimentally, and they found that most of their predictions were accurate. Among the compounds they synthesized were derivatives of two drug compounds that are currently FDA-approved: amoxapine, an antidepressant, and indomethacin, a pain reliever used to treat arthritis.
This computational approach could help pharmaceutical companies predict molecules that will react together to form potentially useful compounds, before spending a lot of money to develop a synthesis that might not work, Kulik says. She and Schindler are continuing to work together on other kinds of novel syntheses, including the formation of compounds with three-membered rings.
“Using photocatalysts to excite substrates is a very active and hot area of development, because people have exhausted what you can do on the ground state or with radical chemistry,” Kulik says. “I think this approach is going to have a lot more applications to make molecules that are normally thought of as really challenging to make.”
More information:
Emily R. Wearing et al, Visible light-mediated aza Paternò-Büchi reaction of acyclic oximes and alkenes to azetidines, Science (2024). DOI: 10.1126/science.adj6771
Provided by
Massachusetts Institute of Technology
Citation:
Scientists use computational modeling to guide a difficult chemical synthesis (2024, June 27)
retrieved 27 June 2024
from https://phys.org/news/2024-06-scientists-difficult-chemical-synthesis.html
This document is subject to copyright. Apart from any fair dealing for the purpose of private study or research, no
part may be reproduced without the written permission. The content is provided for information purposes only.